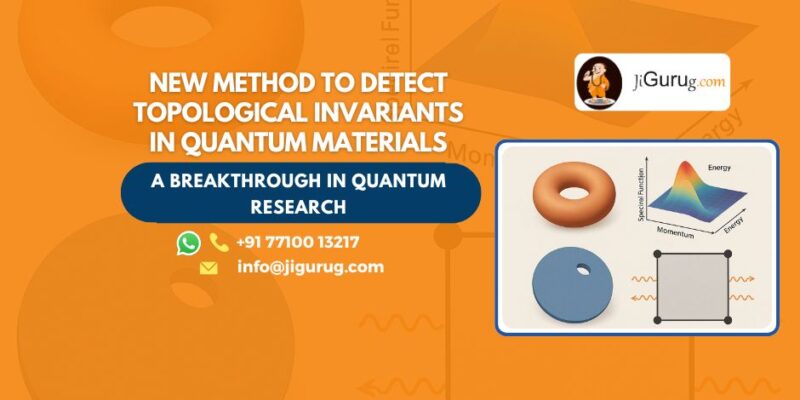
New Method to Detect Topological Invariants in Quantum Materials: A Breakthrough in Quantum Research
In a significant leap for quantum materials science, researchers at the Raman Research Institute have unveiled a groundbreaking method to detect topological invariants—fundamental properties that define the unique behaviour of topological materials. This innovative approach, which utilises the spectral function as a quantum fingerprint, promises to deepen our understanding of quantum materials and accelerate advancements in technologies like quantum computing and energy-efficient electronics.
Understanding Topological Invariants: The Quantum Material’s DNA
Topological invariants are intrinsic characteristics that remain unchanged despite continuous transformations or deformations of a material’s structure. Unlike conventional properties that depend on external factors or appearances, these invariants are rooted in the material’s quantum-level topology. A classic analogy helps to explain this concept: imagine a donut and a coffee cup—both have a single hole, making them topologically equivalent. In contrast, a wada (a doughnut-shaped Indian snack) and an idli (a solid steamed cake) are topologically different because of their distinct hole counts.
At the quantum scale, these invariants govern how electrons and other particles behave within materials, fundamentally influencing their electronic properties. This makes topological invariants crucial for identifying and classifying quantum materials, which exhibit exotic behaviours not found in ordinary substances.
The Importance of Topological Materials in Modern Technology
Topological materials, including topological insulators and superconductors, have attracted immense scientific interest due to their remarkable electronic characteristics. These materials allow electrons to flow along their surfaces without resistance while insulating the interior, a phenomenon controlled by topological invariants such as winding numbers and Chern numbers. These mathematical quantities describe how electron wavefunctions twist and turn across the material’s structure, enabling robust electronic states immune to impurities or defects.
The unique robustness of these states holds immense potential for transformative technologies, including:
-
Quantum Computing: Stable qubits based on topological states can drastically reduce error rates.
-
Spintronics: Devices leveraging electron spin, rather than charge, for faster and more efficient data processing.
-
Energy-Efficient Electronics: Materials that conduct electricity without energy loss could revolutionise power transmission and electronic devices.
The New Method: Spectral Function as a Quantum Fingerprint
Traditionally, detecting topological invariants has posed a considerable challenge. Techniques like Angle-Resolved Photoemission Spectroscopy (ARPES) have been the gold standard for probing electron behaviours in materials. However, these methods can be limited by experimental constraints and do not always provide a comprehensive picture.
The team at Raman Research Institute, led by Professor Dibyendu Roy and PhD researcher Kiran Babasaheb Estake, has pioneered a novel approach using the spectral function—a tool that characterises how energy and particles interact within a material. This function effectively acts as a quantum fingerprint, encoding vital information about the topology of the material.
By analysing the spectral function, researchers can now detect the presence and nature of topological invariants more directly and efficiently. This approach transcends the limitations of traditional techniques, opening new avenues for exploring and classifying quantum materials.
Advantages and Impact of the New Detection Method
This innovative detection technique offers several key advantages:
-
Broader Applicability: Unlike ARPES, which requires complex and expensive equipment, spectral function analysis can be more accessible.
-
Comprehensive Insight: The spectral function captures interactions at a fundamental level, providing richer data on material properties.
-
Enhanced Accuracy: Directly links quantum mechanical behaviour to topological features, reducing ambiguity in identification.
These benefits pave the way for accelerated discoveries in condensed matter physics, enabling scientists to explore previously inaccessible materials and states.
Future Implications: Towards Quantum and Energy-Efficient Technologies
The capability to reliably detect topological invariants is a major milestone that could profoundly impact the future of technology. With this new method:
-
Quantum Computing Development: Improved identification of topological qubits could lead to more stable, scalable quantum computers.
-
Innovative Electronics: Design of novel devices with reduced energy loss and enhanced performance becomes feasible.
-
Advanced Materials Research: Scientists can tailor materials with specific topological properties for customized applications in sensing, communication, and beyond.
As topological materials continue to shape the frontier of science and technology, breakthroughs like this will be crucial for transforming theoretical concepts into practical innovations.
In conclusion, the discovery of a new method to detect topological invariants using the spectral function marks a transformative step in quantum materials research. Spearheaded by experts at the Raman Research Institute, this technique holds promise not only for advancing fundamental physics but also for unlocking the full potential of quantum technologies and energy-efficient systems in the near future.